Astronomers tend to exhaust superlatives when they talk about the ferociously hot and luminous stars known as Wolf-Rayets, which are among the largest, hottest and rarest stars in the universe. Wolf-Rayets are believed to be the final, fleeting stage in the lives of the most massive stars—those starting life with anywhere from 20 to more than 200 times the mass of the sun. These heavyweights are blue and incredibly luminous, burning rapidly through vast reserves of hydrogen fuel with live-fast, die-young abandon. As they burn up, they eject huge amounts of mass in dense, fast winds that flow at astonishing speeds. When they run out of this fuel, these stars collapse under their own gravity in the cataclysmic events we observe as supernovae.
Their extreme nature marks them as celestial outcasts that cluster at the borders of astronomy’s foundational chart, the Hertzsprung-Russell diagram, which maps stars by their brightness and temperature. Wolf-Rayets rise above and beyond the diagram’s “main sequence,” where ordinary stars congregate. They are bloated monsters with surface temperatures that can exceed 200,000 kelvins—30 times hotter than the sun—and radiation fields that can outshine the sun by factors of more than a million.
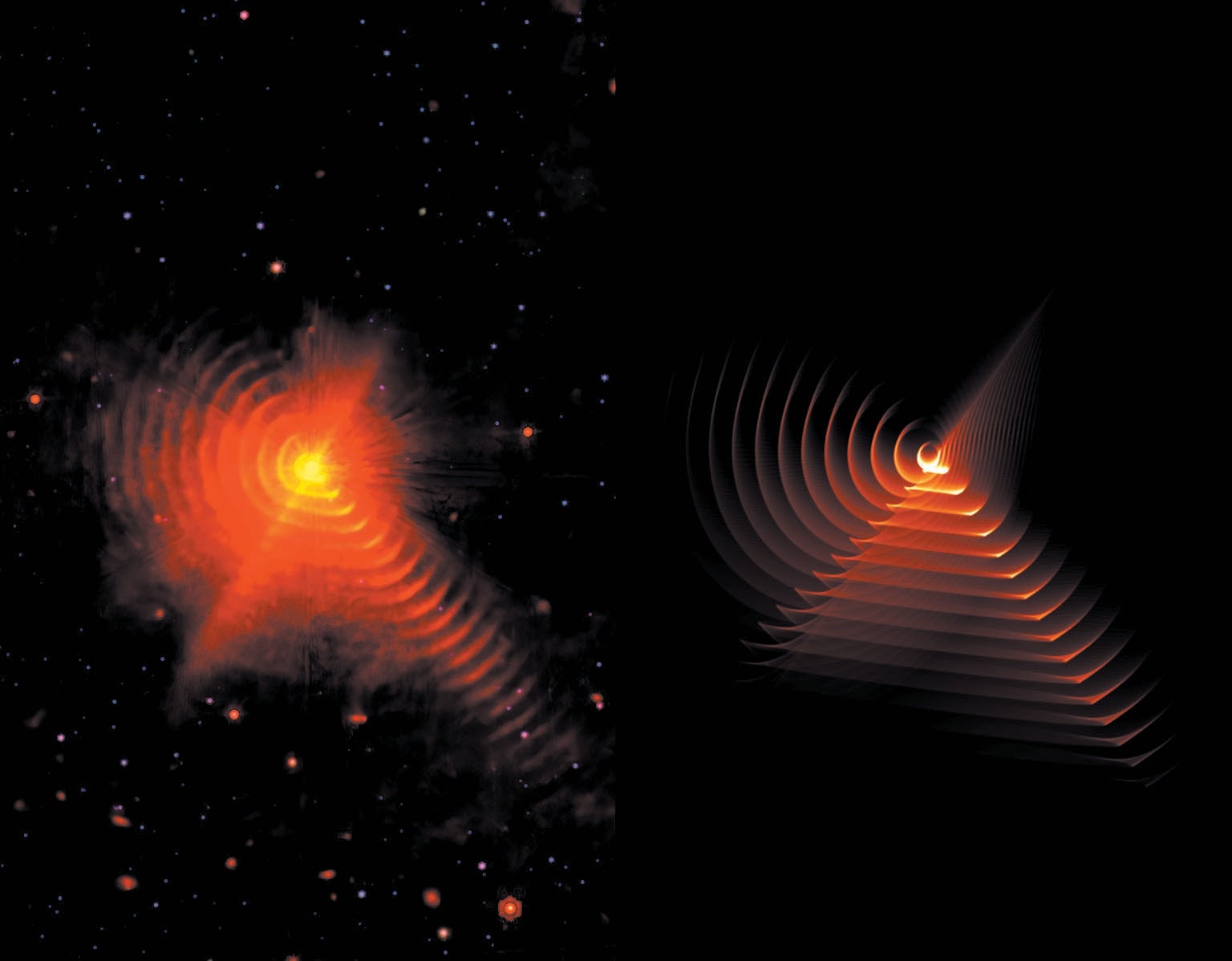
The defining trait of a Wolf-Rayet star—a low abundance of hydrogen—turns out to be a harbinger of doom. After a star exhausts its hydrogen, it will start burning other fuels, such as helium, but this gains the star only a modest stay of execution. Wolf-Rayet lives are measured in millions of years and sometimes much less. This is a blink of the eye compared to our sun’s 10-billion-year life span. And because massive stars are already exceptions among star types, Wolf-Rayets are doubly rare: they are literally one star in a billion. Although their brightness makes them easy for telescopes to find, we know of only a few hundred of them in our entire galaxy.
Despite their rarity, these enigmatic stars have a history of entanglement with the most pressing astronomical questions of the day. As more observations of them arrive from powerful facilities such as the James Webb Space Telescope, this trend is repeating itself. Recently Wolf-Rayets have presented us with new questions about the physics that drives them, which may help solve big mysteries about the nature and fate of stars.
An Enigma Is Born
In 1876, when French astronomers Charles Wolf and Georges Rayet first puzzled over three stars in the constellation of Cygnus, the science of spectroscopy—studying astronomical objects by spreading their light into its constituent colors—was in its infancy. Still, Wolf and Rayet had seen enough normal stars to know that something deeply bizarre was going on. Ordinary stars like the sun have spectra consisting of light from across the range of visible colors, imprinted with a scattering of narrow, fine dark lines that represent wavelengths being absorbed by the chemical elements in the stars. The new stars in Cygnus appeared to be something else entirely: they showed vibrant bands of bright color “more reminiscent of nebulae,” the astronomers wrote, causing them to speculate that these stars might “mainly owe their brilliance to incandescent vapors.”
Over the following decades, astronomers began to better understand the spectra of most stellar types, but Wolf-Rayets still languished as an incomprehensible oddity. They did occasionally ensnare scientists such as Ralph Copeland. In 1884 he made an expedition to the shores of high-altitude Lake Titicaca in Peru, with astronomical equipment packed in by mule train. There he stumbled across the star γ Argus (“gamma Argus,” now known as γ Velorum), whose “intensely bright line in the blue and the gorgeous group of three bright lines in the yellow and orange render its spectrum incomparably the most brilliant and striking in the whole heavens.” Copeland was hooked: “The extraordinary beauty of this spectrum … led me to devote a considerable part of my time to more or less systematic sweeps of the neighborhood of the Milky Way.” He eventually netted another five similar stars. Although none were as spectacular as γ Velorum, this effort more than doubled the catalog of known Wolf-Rayets.
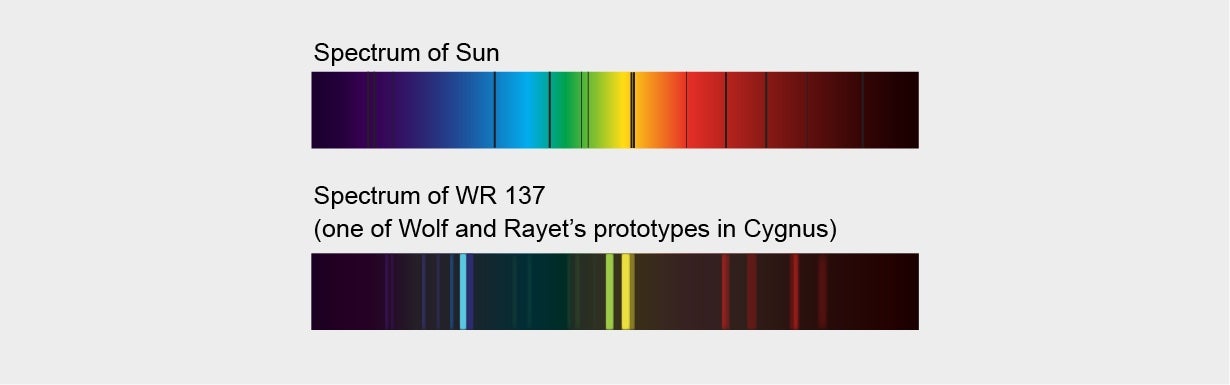
For half a century the Wolf-Rayet phenomenon remained “a door yet unopened and with a key so curious that we are not even sure how to insert it into the lock,” as American astronomer Donald H. Menzel wrote in 1929. But during the 1930s various studies resulted in a gradual understanding of the physics behind the stars. Wolf and Rayet’s comment about “incandescent vapors” was on the right track all along, but astronomers had been reluctant to dial up the physical conditions to the mind-boggling levels required.
The searing temperatures in Wolf-Rayets fuel a radiation field at the stars’ surface so powerful that the light itself becomes a force to be reckoned with. There is a fundamental upper bound to the luminosity of any celestial object beyond which “the radiation observed to be emitted … would blow up the star,” Arthur Eddington wrote in an influential 1926 paper. Wolf-Rayets, it turns out, are so luminous that they flirt with this “Eddington limit,” causing their surface layers to be continually driven off by the stars’ incandescent glare. The key that opened Menzel’s door turned out to be this strong stellar wind, streaming at several thousand kilometers per second—around 1 percent the speed of light. The phrase “solar hurricane” is sometimes used, but this comparison to our sun’s solar wind does not remotely do it justice. Imagine the lightest discernible breath of air on a calm day compared to the force of a powerful water cannon. The divergence between our sun’s solar wind and that of a Wolf-Rayet exceeds that ratio by a factor of more than 10,000.
Even a tiny handful of these overachievers can profoundly impact the ecosystem of an entire galaxy. Streaming winds carry energy, momentum and newly forged elements out into the voids between the stars, blowing bubbles, compressing clouds and heating gas. The most important contribution to the galactic balance from Wolf-Rayet stars is the least expected: stardust. Dust—tiny flakes of star stuff—plays all kinds of crucial roles in the grand cycle of matter in the galaxy, perhaps most of all by shielding and cooling the gas throughout, allowing it to condense to form new generations of stars. Yet astronomers have struggled to account for all the dust they see. In astronomy, dust is a little like snow: plentiful in calm conditions and cool climates. The last place to expect dust creation is somewhere bathed in the hot, harsh ultraviolet radiation surrounding a Wolf-Rayet.
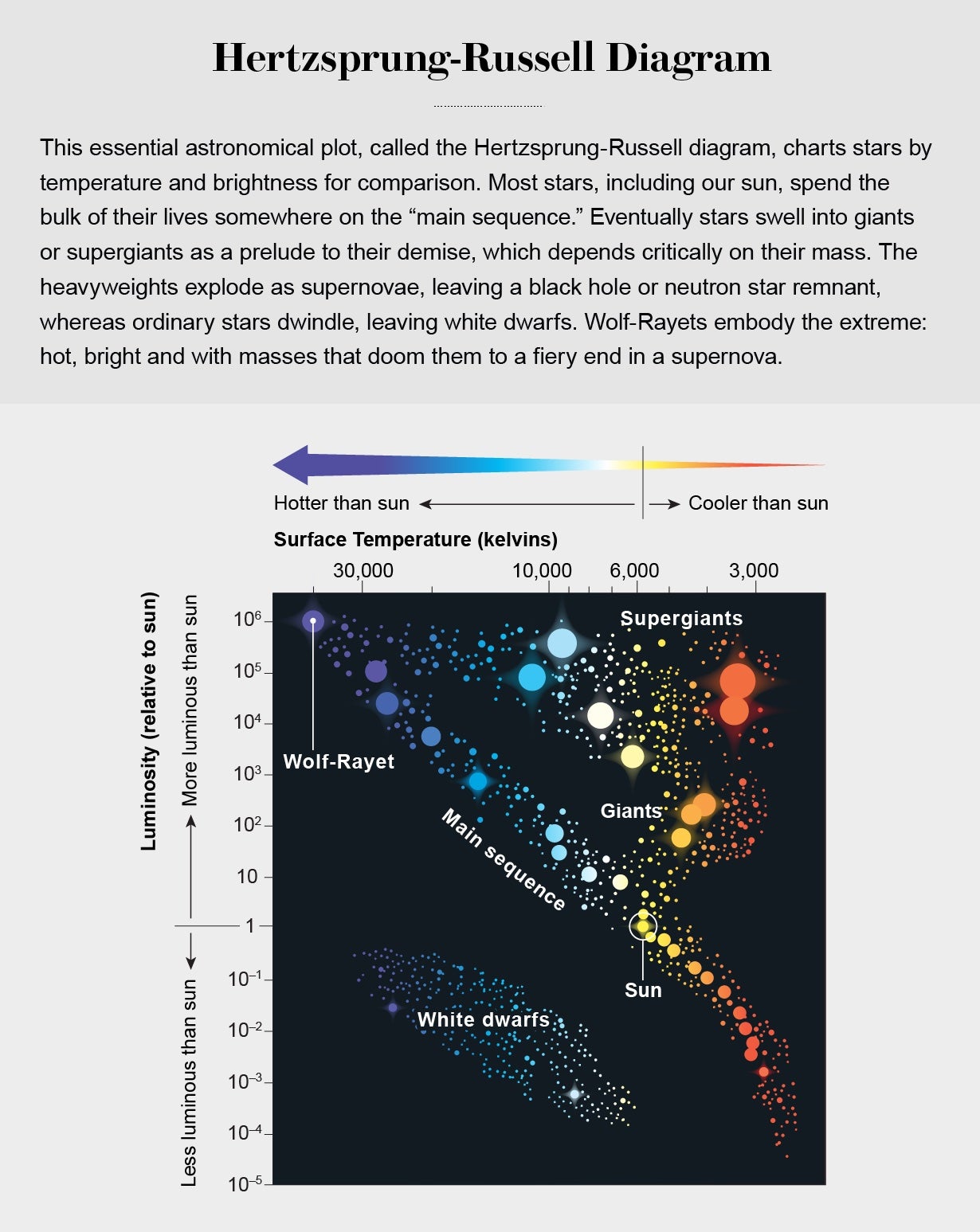
The conundrum of how to form snowflakes in hell was resolved only with the discovery of the miraculous system named WR 140. In the 1980s a team led by Peredur Williams of the Royal Observatory Edinburgh found that dust produced by this star came in pulses spaced eight years apart. The discovery immediately linked the creation of dust to the eight-year period of a binary companion co-orbiting with the Wolf-Rayet. This companion was another luminous blue star on an elliptical orbit. In this binary system, astronomers realized, dust forms when the pair makes its closest approach. As the wind from the Wolf-Rayet collides with and entangles the wind of the massive companion, the two fight each other to a standstill. Here the cool, calm conditions are just right for dust to condense out of the gas. This colliding-wind dust mechanism requires that both stars launch powerful winds—a condition that can be met because massive stars often form along with similarly massive companions.
Unlike WR 140, many other Wolf-Rayets continuously pump out dust, apparently with no regard for the timing of their orbit. Figuring out why, and whether the continuous dust makers work differently from the clockwork dust-created-each-orbit variety, became a key question for my own research.
The Pinwheels
In the mid-1990s I was working in the group of Nobel Laureate Charles H. Townes in California with then student John D. Monnier. The giant Keck Telescopes in Hawaii had just opened for business. To understand Wolf-Rayet dust formation, however, we needed sharp images revealing a level of detail that was beyond the capability of even Keck’s huge 10-meter mirrors. Today we could just switch on an adaptive optics system—now standard equipment that counteracts the shimmering of Earth’s atmosphere. But in the 1990s technology able to image our Wolf-Rayet stars was tens of years and many millions of dollars in the future.
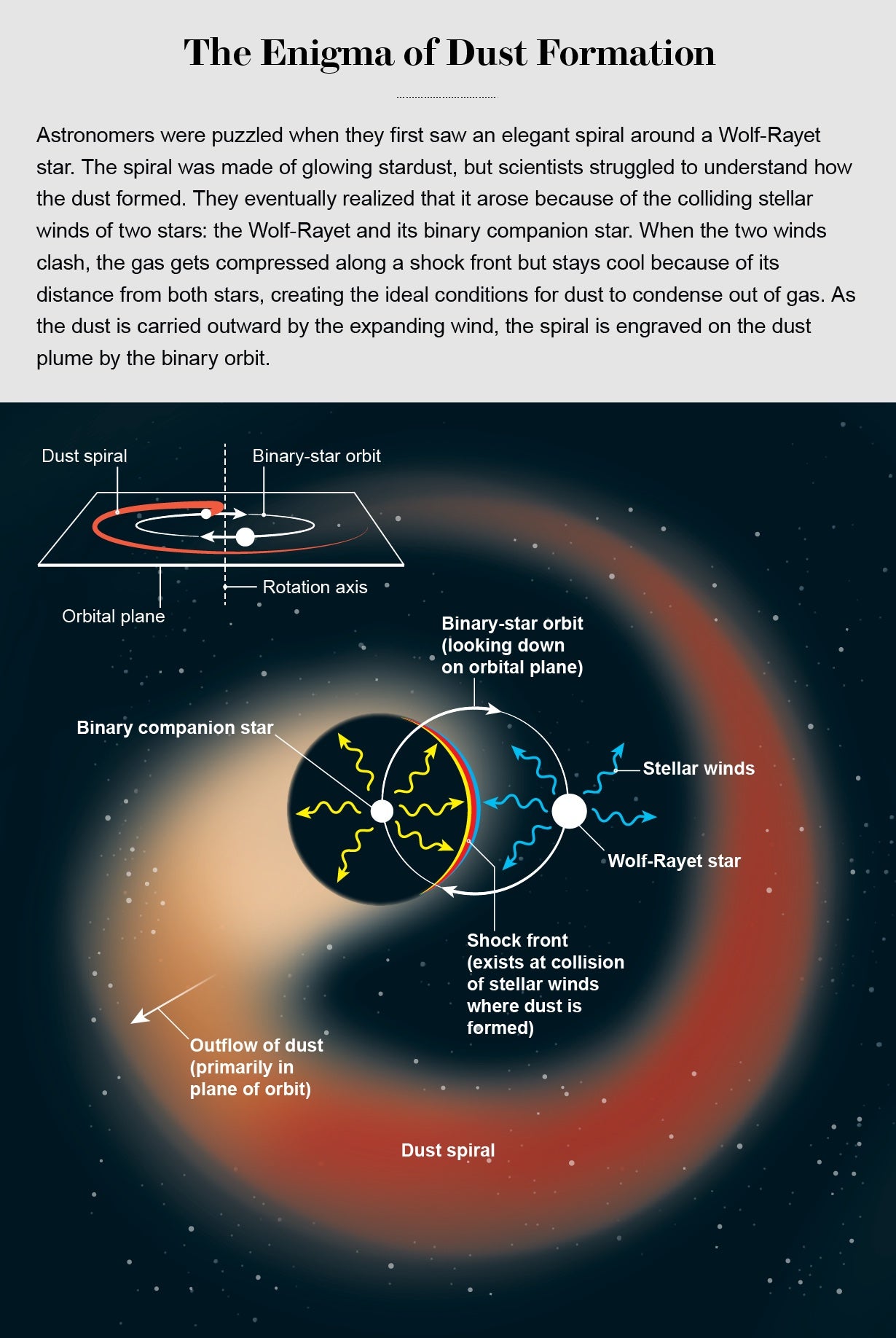
Necessity being the mother of invention, we had no option but to think laterally. We secured a large metal mask, about the size of a trash can lid with carefully arranged perforated holes, to one of the Keck telescopes. By blocking much of the starlight, we transformed the primary mirror into an array of small collectors, allowing Keck to work much like modern radio telescopes that link many smaller antennas together. The gains in image fidelity exceeded our wildest dreams. The entire performance required climbing onto the telescope to swap out masks in the night while perched 15 meters above the observatory floor, which is something I am eternally surprised they ever let us get away with.
Forming images using this technique required significant computer processing, plus a lot of custom code. When we first beheld our most important Wolf-Rayet target, a star designated WR 104, on a computer monitor, it was a shimmering spiral that resembled a weirdly distorted Christmas bauble. I looked at John and groaned, “Never heard of any star shaped like a spiral. How did we get a bug in the code to produce that kind of error?” We went back and improved the code, but the spiral stayed put. It was not until a few months later, when data from a second visit to the Keck telescope produced another spiral, that we accepted reality. The new image was almost the same spiral shape as before but rotated by about 90 degrees. The spiral was real, and furthermore, we had a moving target on our hands.
Hindsight being what it is, I understand now that a spiral is exactly what we should have been looking for all along. What confused us was that dust needs dense, cool gas to form. A Wolf-Rayet can meet only one of these conditions at any given spot: close to the star the gas is dense but hot, whereas far away it is cool but too tenuous. This is where the binary pair comes in. When the winds from the two stars collide, the gas compresses far enough away from the stars for it to stay cool—conditions leading to a “dust nursery.” Dust grains condense out of the gas along a bowl-shaped “shell” where the winds clash. As the stars orbit and their expanding winds sweep outward, the dust spirals out like the jet from a lawn sprinkler.
The result of all this physics manifests as a majestic spiral plume. To the eye of an astrophysicist, however, the beauty is deeper. These structures open a rare window into phenomena we could otherwise never hope to witness. It’s as if nature writes its secrets in a script too tiny to see, but then the expanding wind inflates the text into a giant banner. Here were the properties of the winds, the stars that launched them and the parameters of their orbital dance, laid out for us to read. WR 104 became the prototype for a new class of nebulae that we christened “pinwheels.” We soon found more systems, given names like WR 112 and WR 98a, that shared a common architecture, yet each was unique and distinctly beautiful.
A New Mystery
In the years since, the pinwheels have continued to fascinate, beguile and confound us.
One ongoing puzzle began back in 1963, when the Partial Test Ban Treaty between the U.S. and the U.S.S.R. came into force, prompting America to launch the Vela satellites to monitor compliance by sensing gamma rays given off by nuclear tests. The sensors onboard these satellites began reporting events coming from above, not just below. These so-called gamma-ray bursts have since become one of the hottest topics in astronomy. A subtype of longer-duration bursts, which last more than two seconds, are thought to arise from the supernovae marking the deaths of Wolf-Rayet stars.
Not only are gamma-ray bursts intriguing, but over cosmic time they may even pose a safety risk. Typical supernovae can really affect only their immediate stellar neighborhood. This may not be true of gamma-ray-burst supernovae. Here the energy output is confined to a narrow and powerful beam, so with the right alignment they are visible at vast cosmic distances. Such an alignment for a nearby event may herald danger.
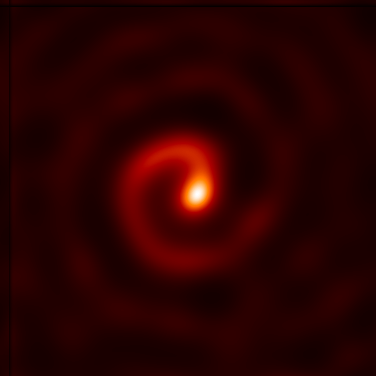
Speculative studies have suggested that events in Earth’s fossil record, such as the Late Ordovician mass extinction, could have been caused by a gamma-ray-burst strike. The risk of such a cataclysm exists only when Earth is situated exactly along the line of the burst. For the first time our data allowed us to analyze the likely axis of a possible future burst from our pinwheel Wolf-Rayets. Unfortunately, WR 104 might be pointing our way.
Yet the statistical threat posed by a future gamma-ray strike from WR 104 is truly minuscule: several very unlikely things would have to happen all in sequence, including the low-probability event WR 104 can host a gamma-ray burst (rather than a typical supernova) in the first place. When writing up our research, my colleagues and I weighed the vanishingly small but nonzero odds—and the fact humanity faces more serious threats from things such as climate change—and decided to include only a few short, carefully worded sentences on this possibility in our paper. Of course, these lines immediately went viral on the Internet. Soon I was in my department head’s office, explaining how I’d become famous for 2012 end-of-the-world Mayan calendar conspiracy theories.
More recently, we’ve recovered spectacular new data on the pinwheels from observatories such as the James Webb Space Telescope, the likes of which Wolf and Rayet could hardly have imagined 150 years ago. Among the very first JWST images was a revelatory vision of an old friend, WR 140 (of the eight-year dust cycle mentioned earlier).
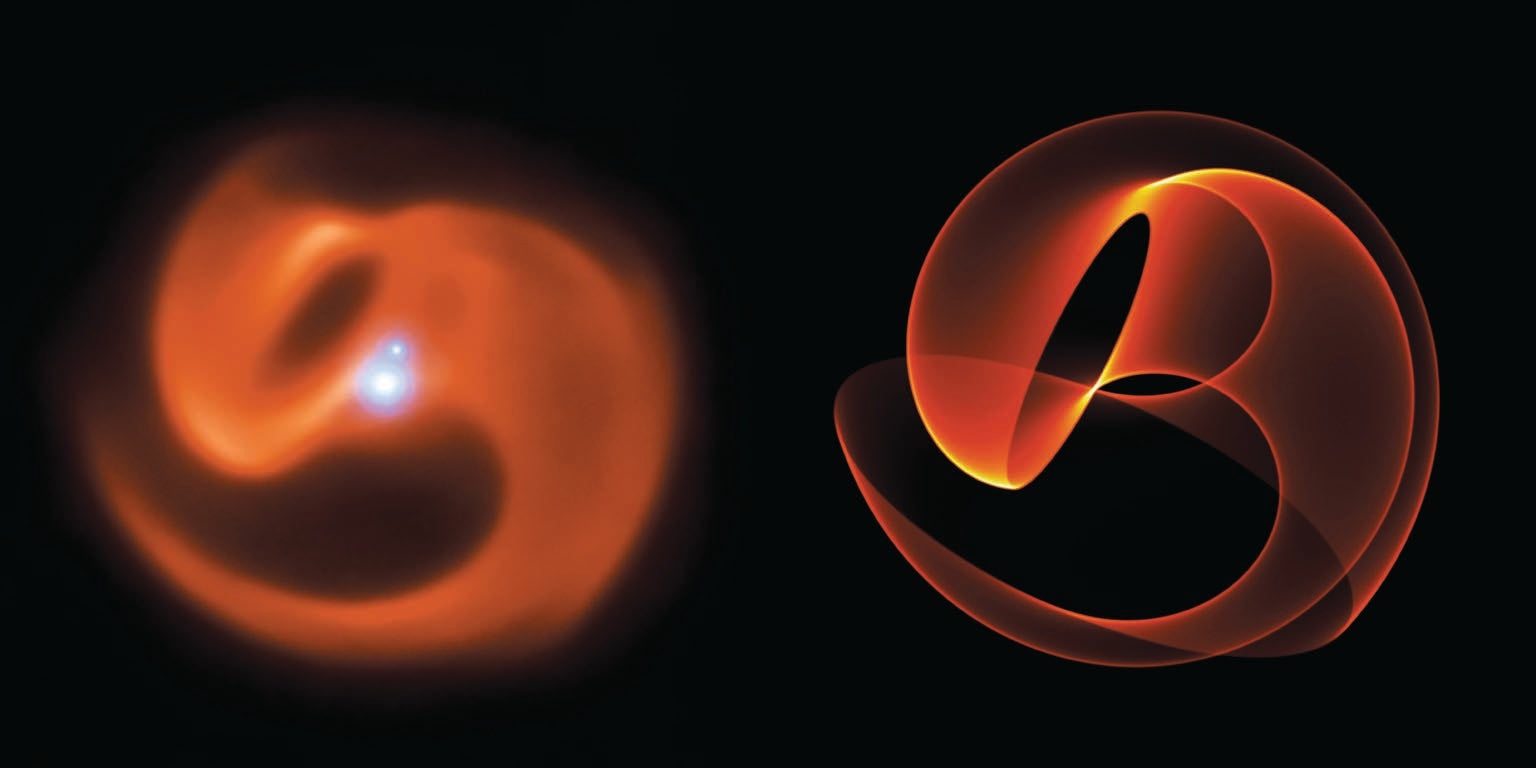
With the staggering leap in sensitivity from this new observatory, we can see shell after shell of dust—nearly 20 of them marching out into space, each an exquisitely sculpted replica nested within the older, more inflated one preceding it. My student Yinuo Han and I compared this observation with a previous computer model we’d built to describe only WR 140’s single innermost dust shell. When we extrapolated out to see what 150 years of repeat shells might look like, our result almost perfectly mimicked the onion-layer image from JWST, showing the uncanny power of mathematics to echo the real world.
Perhaps the most exciting of the new discoveries has been the first confirmed twin Wolf-Rayet binary, a system called Apep, which my colleagues and I named after the mortal enemy of Egyptian sun god Ra. Images of the system evoke the mythology, suggesting a star embattled within a serpent’s coils. Apep also offers a surprise. Our calculations clock the speed of the Wolf-Rayet’s expanding gas wind, as well as the expansion rate of the dust. These two numbers should agree, and for all the other pinwheels, they do. In Apep, however, the dust streams out only one third as fast as the gas yet is caught in the teeth of the strongest howling gale known to stellar physics. It’s like finding a feather adrift in a hurricane, somehow floating along at its own gentle pace. How does dust around Apep perform this magic trick? Nobody knows for sure.
Once again, Wolf-Rayets are humbling astronomers who think they understand how things work. And by the time we have the answer to this question, I’m sure these enigmatic stars will have given us still deeper mysteries. They have a history of mixing things up every time they make an appearance.