What is life? Like most great questions, this one is easy to ask but difficult to answer. Scientists have been trying for centuries, and philosophers have done so for millennia. Today our knowledge is so advanced that we can precisely manipulate life’s building blocks—DNA, RNA and proteins—to build biological machines and engineer new genomes. Yet despite all we know, no universal consensus currently exists on life’s fundamental definition.
The reason life’s definition still eludes us is simple: we know of just one type of life—the kind that exists on Earth—and it’s challenging to do science with a sample size of one. This is the so-called N = 1 problem (wherein “N” denotes the number of eligible candidates that scientists can study). No matter how ingenious researchers may be in divining life’s general principles from the single instance of which they’re sure, they have no way of confirming whether they’ve done so successfully until N increases. Searching for another instance of life—whether it’s found right here on Earth, elsewhere in the solar system or beyond—is one way to expand N. The search has scarcely begun, but it has already consumed billions of dollars and countless hours of labor, even though there is no guarantee that a discovery will ever come.
There is, however, another potential way to solve the N = 1 problem: instead of finding some second genesis for life, some scientists are seeking to create one. The field of artificial life—called ALife for short—is the systematic attempt to spell out life’s fundamental principles, either by studying lifeless natural systems that exhibit lifelike behavior or by building artificial systems to compare against nature’s creations. Many of these practitioners, so-called ALifers, think that somehow making life from scratch is the surest way to really understand what life is—an approach perhaps best summarized as “build first, explain later.”
Spoiler alert: so far no one in this nascent domain has convincingly made artificial life—though not for any lack of trying. This demonstrably dismal track record makes ALife a ripe target for criticism that ranges from accusations of “playing God” to declarations of the field’s dubious scientific value.
Takashi Ikegami, a complexity scientist at the University of Tokyo, is tired of such complaints. His field is just like any other basic science that seeks knowledge for knowledge’s sake, so asking about “the point” of ALife might be, well, missing the point entirely, he says.
“The existence of a living system is not about the utility of anything,” Ikegami says. “Some people ask me, ‘So what’s the merit of artificial life?’ Do you ever think, ‘What is the merit of your grandmother? What is the merit of your dog?’”
Endless Evolution
As much as many ALifers detest emphasizing their research’s applications, the quest to create artificial life could have practical payoffs, too. Artificial intelligence may be considered ALife’s more glamorous cousin in that researchers in both fields are enamored by a concept called open-ended evolution. This is the capacity for a system to create essentially endless complexity, to be a sort of “novelty generator.” The only system known to exhibit this is Earth’s biosphere—an ongoing, multibillion-year evolutionary explosion of biodiversity that ultimately traces back to simple, single-celled ancestral organisms. If—or when—the field of ALife manages to replicate life’s inexhaustible “creativity” in some virtual model, presumably those same principles could give rise to truly inventive machines.
Currently, in AI, “you can build these monstrous deep-learning systems, but at some point, these systems can’t learn anymore,” says Steen Rasmussen, an ALife researcher and physicist at the University of Southern Denmark. “What does it take for a system to continue to learn? Nobody knows.”
Compared with the developments of AI, advances in ALife are harder to recognize. One reason for the discrepancy is that ALife is a field in which the central concept—life itself—is vexingly undefined. The lack of consensus among ALifers doesn’t help either—there isn’t a set of shared tenets to guide their collective work, let alone standards to evaluate it. The result is a diverse but meandering array of projects that each advance haphazardly along their unique paths. For better or worse, ALife mirrors the very subject it studies. Its muddled progression is a striking parallel to the eons-spanning evolutionary struggles that have so profoundly shaped Earth’s biosphere.
Assembling ALife
ALife’s unofficial kickoff came in 1987 at the first Interdisciplinary Workshop on the Synthesis and Simulation of Living Systems at Los Alamos National Laboratory, where the field gained the legitimacy of a name. Coined by computer scientist Christopher Langton, the term “artificial life” provided a unifying label for the scattered interdisciplinary studies of scientific misfits and vagabonds pondering lifelike behavior. Rasmussen was one of the workshop’s attendees 36 years ago. He recalls feeling like he was “coming home.”
Some ALife ideas date further back. In 1948 mathematicians John von Neumann and Stanislaw Ulam set out to formulate how machines could, in theory, self-replicate—a trait that ALifers later targeted as a hallmark for life. Applying pen to paper, the mathematicians constructed the concept of cellular automata, dynamical entities made up of shaded or unshaded cells skipping across a two-dimensional grid. In von Neumann and Ulam’s formulation, each individual cell blinks on or off based on simple relationship rules with its neighbors. Depending on the initial placement of the constituent cells, their clusters can showcase surprisingly complex behaviors such as self-replication ad infinitum. The mathematicians’ work with cellular automata helped them realize that replicating living cells must be able to somehow internalize and record information about their environment—an insight prescient to the then-growing recognition of DNA’s role as earthly life’s information-storage molecule.
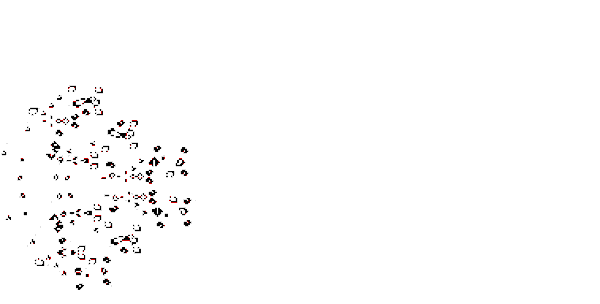
The link between these cellular automata and the pursuit of artificial life grew stronger when, more than two decades later, mathematician John Conway designed the Game of Life. First popularized in the October 1970 edition of Scientific American, this was a concept for a game that expanded on von Neumann and Ulam’s rules. Soon versions of Conway’s game were running at scale on early digital computers, allowing easier explorations of the intricate patterns and complex behaviors that emerged. The Game of Life was a hit, and it lured players worldwide to become armchair experimentalists in their hunts for new digital “organisms” among the constellations of pixels flickering across their screens.
From humble beginnings, three distinct branches of ALife ultimately arose. So-called “soft” Alife, such as the Game of Life, simulates life in silico. Another branch known as “hard” ALife involves the creation of autonomous robots. The third branch, “wet” ALife, concerns the creation of synthetic organisms using known principles of biochemistry.
Of these, hard ALife trails the other branches in the race toward a second genesis. To date, no robot can spontaneously assemble itself without outside assistance from humans or other machines.
Software-based organisms, examples of soft ALife, are much further along. Some of them can evolve and compete for spatial and temporal resources on the computer that hosts them. One example is the virtual universe Tierra, developed by ecologist Thomas Ray, which features entities that replicate, mutate and compete against one another from generation to generation. But this virtualized “survival of the fittest” still fails to fulfill the criterion of open-endedness: the complexity and novelty of digital organisms in Tierra and its ilk eventually plateau.
Chemical-based wet ALife research hews closest to real biology and typically garners the most scientific respect. For instance, in a series of experiments in the 2010s, geneticist J. Craig Venter and his team successfully transplanted synthetic genomes into hollowed-out Mycoplasma bacteria to create designer self-replicating bacterial cells. In 2021 a different team of scientists fashioned clumped frog cells into “xenobots” that could swim around within their petri dishes. These mobile blobs could also group scattered frog cells together to form new xenobots—another demonstration of self-replication.
A Learning Experience
More recently, hybrid approaches have emerged that focus on smaller pieces of the “life” puzzle. For example, a study published last September combined wet and soft ALife by simulating how simple chemical systems can exhibit learning. Learning is a hallmark of life because it allows organisms to survive environmental curveballs. Evolution on the larger scale of species and ecosystems can be thought of as learning via selective adaptation to shifting habitats. Performed by California Institute of Technology physicist Stuart Bartlett and French programmer David Louapre, the work showed how only a handful of virtual chemical reactions could constitute both long- and short-term memory for simple simulated mixtures of chemicals seeking protection from recurring doses of “toxins.” After repeated poisonings, the chemical systems could “learn” to nail down the timing of manufacturing an “antidote”—wielding it both preemptively and post hoc—to survive the chemical threat.
The research is “really nice work,” says Rasmussen, who wasn’t involved in the study. This, he says, is because it pushes back the threshold at which certain underpinnings of cognition might appear in life’s emergence. “We didn’t know that [it] was possible” for such complex behavior to arise in such spartan conditions, he adds.
Bartlett and Louapre might have demonstrated the simplest form of chemical learning to date, but they haven’t identified how a nonlearning system could start learning in the first place, says Nicholas Guttenberg, a freelance machine-learning research consultant who also was not involved in the research. The capacity to “learn,” he points out, was something that Bartlett and Louapre had to preprogram into their simulation. That raises an obvious question, he says: “Will learning spontaneously emerge without a hand-designed relationship first being put in?”
Such questions have not discouraged Bartlett, who argues that his virtual chemical mixtures are minimally alive. They dissipate free energy, grow exponentially, stabilize internal processes and learn about the environment—all criteria for Bartlett’s personal working definition of “life.” They even replicate—but they don’t deviate from generation to generation, so they cannot enable any lifelike runaway rise in complexity.
Existential Crisis
Even if open-ended evolution is the last lingering box to check off for creating artificial life, the community’s efforts may be doomed, says Stuart Kauffman, a biochemist at the Institute of Systems Biology in Seattle. Kauffman is seen as one of ALife’s founders, but he now believes achieving open-ended evolution is an insurmountable hurdle for the field because ALife research is fundamentally incompatible with the scientific method.
Science, Kauffman says, proceeds by logical deduction to generalize gathered observations to an overarching theory. But unpredictability is the essence of life’s open-ended evolution, so science’s analytic approach can’t anticipate a species’ evolutionary trajectory in the wild beforehand, he says.
In evolutionary biology lingo, life is said to “exapt,” which means it can co-opt preexisting components for new functions. Swim bladders that control buoyancy in modern-day fish are thought to have evolved from the lungs of ancient ancestors. Feathers that kept prehistoric birds warm were gradually repurposed for flight and mating displays. “Anything has indefinitely many possible uses,” Kauffman says. The problem of predicting how evolution will spin one feature toward its next purpose isn’t a matter of rolling dice; it’s a matter of predicting when someone will come along and throw the dice to break through a nearby window instead.
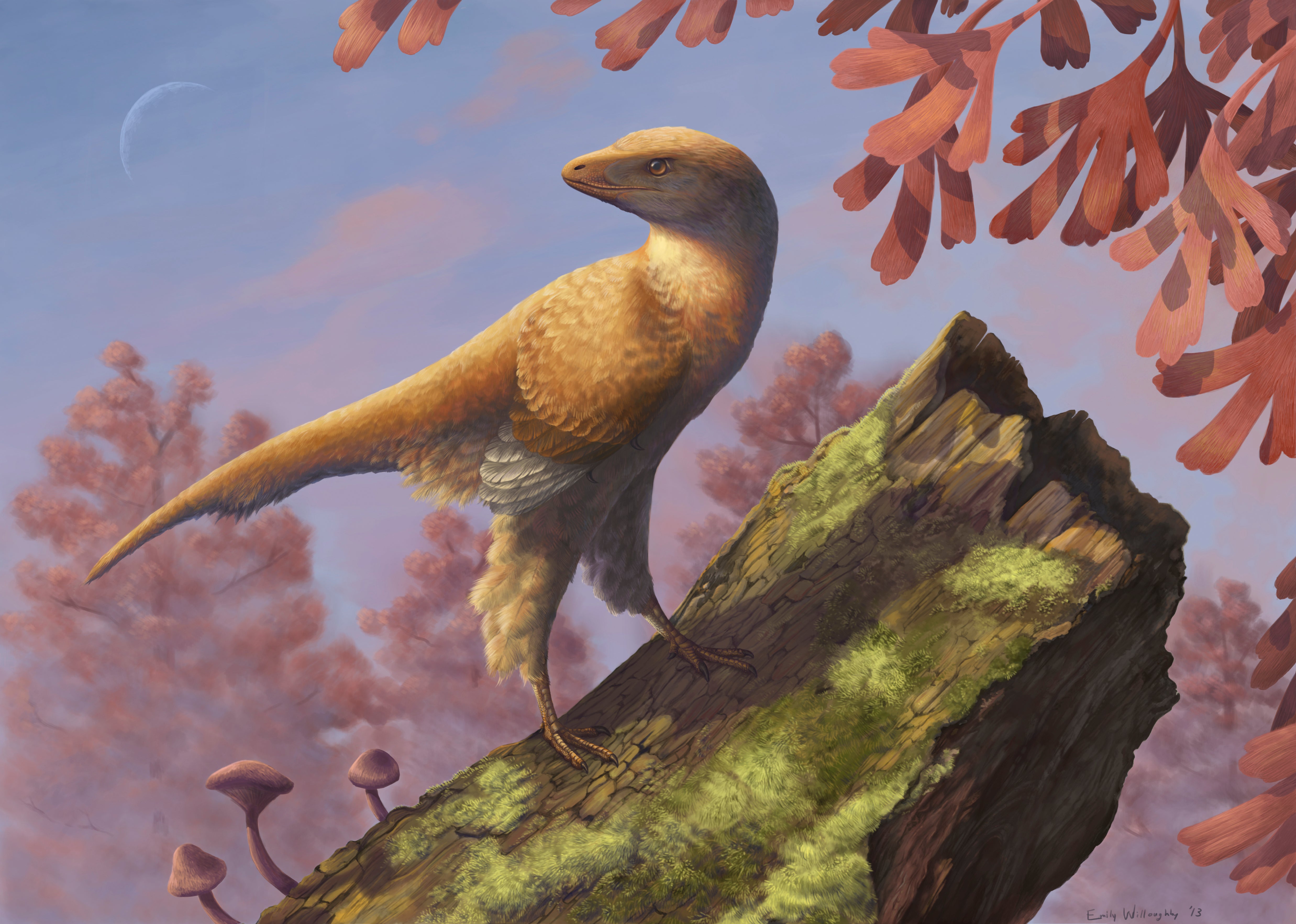
ALife efforts potentially have another fatal flaw, says Carol Cleland, a philosopher at the University of Colorado Boulder. ALife’s build-to-understand approach cannot increase N, she maintains, because every product rests on assumptions that hew too closely to earthly life as their sole reference. Attempts at another genesis may capture several aspects of lifelike behavior, but none can be unquestionably alive or a novel instance of life built from scratch.
Assembled from biological parts and guided using familiar biochemistry principles, wet ALife’s living creations aren’t original life-forms, Cleland contends—they’re merely unnatural ones. As such, they don’t tell scientists about other possibilities for life. “It’s basically like taking apart a car and then putting it back together by replacing some of the metal parts with plastic parts and saying, ‘Look, I created an alternative car!’” she says.
Hard and soft ALife run the opposite problem of being too far removed from flesh and blood. Their builders often assume that the definition of life transcends an organism’s material form and is instead embodied in its functional properties—and who’s to say that is a correct strategy for generalizing life in the first place? Moreover Cleland argues that it’s always possible to come up with an abstraction of life so coarse that it inadvertently includes some examples of nonlife. Calling out the hubris in scientists who claim to know which characteristics are key and universal to all life from just N = 1 isn’t pessimistic sniping, she says—it’s a pragmatic statement of fact.
“You can explore certain characteristics of known life,” Cleland says. “But you don’t have any guarantees that those characteristics are necessary for life.”
In Cleland’s conception, hard and soft ALife are too abstract, whereas wet ALife is too concrete. “You need a level of abstraction in between to really understand the nature of life,” she says. The need for a middle way to bridge these gaps is not lost on most ALifers, but that doesn’t mean they know how to find it from a single case of N.
Saving Grace
If anyone can restore faith in ALife research, perhaps it’s Chris Kempes, a mathematical biologist at the Santa Fe Institute. His strategy for extracting universal laws from the N = 1 of biological life is to run detailed statistical analyses of Earth’s biodiversity. Four billion years or so of open-ended evolution has generated extensive variation across the biological world that reveals recurring relationships among its members and allows scientists to surmise shared constraints.
But one must be careful when pinpointing life’s common grounds. It would be naive to look at DNA present in all biological life and conclude that it’s necessary for all life. The key is “finding the right abstractions” that are neither too specific nor too general, Kempes says, echoing Cleland’s concerns.
To thread that needle, Kempes seeks to link such abstractions back to fundamental physical principles and quantities. Abstractions that have a physical basis will rightfully be laws of life and should hold up among any N anywhere in the universe, he says. This approach may turn open-ended evolution—maligned as ALife’s curse—into its savior.
Kempes and his like-minded ALife peers have logically inferred some obvious constraints on life, such as energy, for example, “because it’s the budget for everything an organism wants to do,” he says. Scientists have long known that organisms’ metabolic rates tend to increase as a fraction of body mass. Explanations for the energy-mass relationship range from maintaining body temperatures to achieving an efficient circulatory system. “There’s a well-defined optimum in that space,” Kempes says. “I think that’s a law of life.”
This energy-mass relationship is low-hanging fruit, but other elements of a universal theory may not be so recognizable. “I do think we’ll have a complete theory of life, although I don’t know exactly what it’ll look like,” Kempes says. “I think it’s going to take the field a while to understand how long that list of the laws of life is.”
In Its Infancy
ALife’s fuzzy nature is characteristic of any scientific discipline in its infancy, its practitioners contend. “ALife is preparadigmatic,” says Sara Imari Walker, an astrobiologist and theoretical physicist at Arizona State University. For some, this embryonic state only adds to the urgency of engaging with ALife now. The field’s open-endedness is both a challenge and opportunity—fallow-but-fertile ground for germinating ideas that could blossom to shape research for generations to come.
Undefined and unconstrained, ALife drives its adherents to repurpose old ideas and generate novelty. The three branches of its metaphorical “tree”—hard, soft and wet—have not yet grown to towering, divergent heights, which allows practically anyone to climb between them. In this view, the product of collaborative work between a scientist such as Bartlett and an ALife newcomer such as Louapre is not so much a research paper as it is a potent hybridization event, one that is analogous to the evolution of symbiotic species that work together toward common survival—and, maybe someday, dominance.
It may be, of course, that these characteristics aren’t in any way surprising or singular. They may apply universally to all complexity-steeped acts of evolution. Ultimately ALife may be nothing special. But even this dismissal suggests something strangely humbling yet grandiose: perhaps, just like life itself throughout the cosmos, the rise of ALife will prove inevitable.